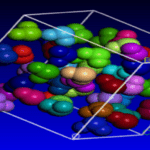
Data-Driven Molecular Design in Chemical Engineering
مهر ۷, ۱۴۰۳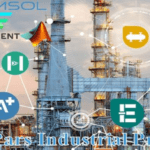
Industrial and Semi-Industrial Project
مهر ۷, ۱۴۰۳Introduction
Climate change is one of humanity’s greatest challenges in the 21st century. The emission of greenhouse gases, particularly carbon dioxide (CO2), plays a major role in this phenomenon. CO2 electroreduction emerges as a sustainable energy conversion process that offers an innovative solution for converting CO2 into valuable products such as synthetic fuels, chemicals, and polymer feedstocks. This promising process paves the way for transforming carbon dioxide into carbon-neutral fuels and valuable chemicals. However, it faces challenges such as low efficiency and high costs.
Miniaturized Membrane Reactors
Miniaturized membrane reactors are a new type of electrolytic reactor that use porous membranes to separate the products of CO2 electroreduction from the electrolyte solution. This approach offers numerous advantages, including:
– Increased efficiency: Membranes can enhance product concentration in the electrolyte solution, leading to reduced energy consumption and improved process efficiency.
– Selectivity: Membranes can be employed to selectively separate specific products of CO2 electroreduction, such as carbon monoxide (CO) and methane (CH4).
For instance, the costs of membranes and operational processes can be high, and the long-term stability of reactors under real operational conditions has yet to be fully demonstrated. Nevertheless, there are ample opportunities to overcome these challenges and develop cost-effective and sustainable miniaturized membrane reactors for CO2 electroreduction. These reactors have the potential to become a key technology for CO2 electroreduction and greenhouse gas emission reduction.
Method
In this study, researchers designed and constructed a new miniaturized membrane reactor for CO2 electroreduction using stereolithography-based 3D printing (SLA). The module must include a cavity for placing the counter electrode in the anode chamber and another cavity in the cathode chamber for the working electrode. Each chamber should have two additional cavities for a miniaturized reference electrode and a pH sensor.
The reactor must include inputs for reagent injection and outputs for removing it. It should be designed as small as possible to maximize reagent consumption and the reagent-to-catalyst surface ratio. After designing and printing the reactor, electrode holders were also created using SLA technology with Form 3 clear resin (Formlabs). The 3D designs created with FreeCAD were sliced using the Preform software. After printing the reactor, uncured resin was removed by washing with isopropanol and subsequently cured with UV light.
Results
Laboratory results indicated that the new miniaturized membrane reactor significantly enhances the efficiency of the CO2 electroreduction process. This improvement stems from reduced electrical resistance and additional potential required to initiate the reaction due to the shorter distance between electrodes. The findings of this study demonstrate that new miniaturized membrane reactors can serve as a promising solution to overcome the efficiency and cost challenges of the CO2 electroreduction process.
These reactors can facilitate increased production of valuable products from CO2 while helping to reduce experimental costs and optimize reaction conditions. This study indicates that the new miniaturized membrane reactors possess considerable potential for advancing the CO2 electroreduction process, making it a cost-effective and sustainable technology for reducing greenhouse gas emissions and producing valuable products.
Advantages of 3D Printed Reactors in CO2 Electroreduction Process
Studies on CO2 electroreduction performance highlight the superior capabilities of 3D printed reactors. This superiority arises from reduced spacing between electrodes, leading to lower electrical resistance and reduced energy and material consumption in each experiment. However, the overall Faradaic efficiency did not significantly increase with the 3D printed reactor.
The natural oxide layer on tin foil also plays a vital role in the CO2 electroreduction process. To avoid product interference in future studies, the use of thicker proton exchange membranes may be necessary.
Reactor Modeling in COMSOL
3D printing, or additive manufacturing, has revolutionized various industries by enabling the creation of complex geometries that are challenging to produce otherwise. One significant application of 3D printing in reactor construction is in the chemical and pharmaceutical industries. COMSOL Multiphysics, a powerful finite element analysis (FEA) software, provides an integrated environment for modeling and simulating the performance of these 3D printed reactors.
Key Features of COMSOL for Reactor Modeling
- Multiphysics Integration: COMSOL excels in simulating coupled physical phenomena, crucial for reactor modeling. This allows users to integrate fluid dynamics, heat transfer, chemical reactions, and structural mechanics into a single model.
- Geometry Handling: This software can directly import complex geometries from CAD software, making it ideal for working with intricate 3D printing designs and ensuring that the model accurately reflects the physical reactor.
- Customizable Physical Interfaces: Users can create custom physical interfaces using the COMSOL Application Builder, which is particularly useful for unique reactor designs and new 3D printing materials.
- Parametric Studies and Optimization: COMSOL supports parametric studies and optimization investigations, enabling exploration of various design parameters and operational conditions to improve reactor performance.
Modeling Process in COMSOL
- Geometry Creation and Import: The initial step involves creating the reactor geometry using COMSOL’s built-in tools or importing it from external CAD software. This geometry represents the 3D printed reactor and includes all internal and external features.
- Material Properties: Defining the material properties of reactor components is critical. COMSOL’s material library includes a wide range of materials, and users can also define custom materials, including specialized 3D printing materials like polymers or metal alloys.
- Physics Setup: Users then select appropriate physical interfaces. For reactor modeling, this typically includes:
– CFD (Computational Fluid Dynamics): For simulating fluid flow within the reactor.
– Heat Transfer: To consider thermal effects, especially in reactors where exothermic or endothermic reactions occur.
– Chemical Reaction Engineering: For modeling the kinetics of chemical reactions taking place inside the reactor.
4. Mesh Generation: Proper mesh generation is critical for accurate simulation results. COMSOL offers various meshing tools capable of handling the complex geometries common in 3D printed reactors.
5. Simulation and Post-Processing: Once the model setup is complete, simulations are run to solve the governing equations. Results are then analyzed using COMSOL’s powerful post-processing tools, which can visualize temperature distribution, concentration profiles, fluid velocity, and more.
Case Studies and Applications
Case Study 1: Microreactor for Chemical Synthesis
One study demonstrated the use of COMSOL to model a 3D printed microreactor designed for chemical synthesis. The reactor’s small size and complex internal channels were easily managed with COMSOL’s geometry import and meshing capabilities. The simulation focused on optimizing flow rates and reaction conditions to maximize yield and efficiency.
Case Study 2: Bioreactor for Cell Culture
Another application included modeling a 3D printed bioreactor used for cell culture. COMSOL’s multiphysics capabilities allowed for simultaneous simulation of fluid dynamics, nutrient transport, and cell growth. This holistic approach enabled researchers to optimize bioreactor design for improved cell viability and productivity.
Conclusion
3D printing, due to its advantages such as cost-effectiveness, rapid manufacturing, and the ability to create complex parts without expensive workshops or specialized labor, is a suitable alternative to traditional CNC techniques in prototyping. Thus, in most cases, after achieving the final prototype and preparing for commercialization or mass production, traditional workshop methods demonstrate significant superiority. In other words, these two methods complement each other, each having its specific applications.
Both 3D printing and traditional mass production methods have specific advantages and applications. 3D printed reactors have advantages in energy and material consumption, but there is a need for advancements in Faradaic efficiency and proton exchange membranes. Automated testing systems with 3D printing can accelerate research processes and improve result reproducibility.
APIPCO, leveraging its expertise and experience, is capable of conducting modeling of 3D printed reactors in the COMSOL software environment.