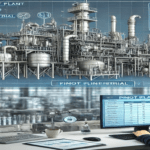
Exploitation of laboratory pilots in industry
دی ۷, ۱۴۰۳Introduction
In industrial processes, the design and construction of various equipment for the production stage are of paramount importance. This equipment, including reactors, towers, mixers, scrubbers, and cyclones, plays a vital role in optimizing performance, reducing costs, and increasing efficiency. Before introducing this equipment into the production line, initial tests are conducted on a smaller scale in laboratories to accurately assess their performance and functionality.
Semi-Industrial and Laboratory Scale projects constitute the initial stages of the design and development of this equipment. At these stages, engineers can simulate process conditions and identify potential problems before the equipment is scaled up. These processes allow engineers to test complex processes in a controlled environment and on a smaller scale, thereby reducing potential risks and challenges.
This article examines the design and performance of key equipment in various industries on a laboratory and pilot scale
Construction of Industrial Equipment on a Laboratory and Pilot Scale
The process of constructing industrial equipment on a laboratory and pilot scale involves several key stages that enable engineers to evaluate equipment performance before mass production. These stages are as follows:
Initial Design:
In this phase, initial ideas for the desired equipment are developed. Engineers create a conceptual design based on process requirements and technical specifications. This design includes the selection of materials, dimensions, and performance characteristics.
Simulation:
After the initial design, simulation software is used to predict the equipment’s behavior under various conditions. These simulations help identify design weaknesses and strengths and allow for modifications before construction.
Prototype Construction (Laboratory Scale):
A small-scale prototype of the designed equipment is constructed. This prototype is carefully prepared for testing under controlled conditions. The goal of this stage is to evaluate the actual performance of the equipment physically.
Testing and Evaluation:
The prototype undergoes various tests to evaluate its performance and efficiency. The results of these tests help identify problems and the need for modifications.
Final Design:
After evaluating the prototype, the final design is revised based on the feedback obtained from the tests. This design must be suitable for large-scale construction.
Pilot-Scale Construction:
In the next stage, the equipment is constructed on a pilot scale. This equipment is designed to be similar to the final full-scale version. The goal of this stage is to test the equipment’s performance under near-real production conditions.
Real-World Testing:
The pilot-scale equipment is subjected to real-world operating conditions. These tests help identify potential challenges and problems in mass production and ensure that the equipment is ready for market.
In summary, constructing industrial equipment on a laboratory and pilot scale is a systematic and precise process that allows engineers to prepare equipment for large-scale production with greater confidence. These stages help reduce risk and optimize the design and production process. In the following sections, we will examine several key industrial equipment and their evaluation in laboratory and pilot-scale conditions
Design and Construction of Distillation Columns on a Laboratory and Pilot Scale
Distillation columns are key equipment in chemical separation processes used in various industries, including oil, gas, and chemicals. The design and construction of these columns on a laboratory and pilot scale involve specific stages, which are discussed below.
Design and Construction of Distillation Columns on a Laboratory and Pilot Scale
Distillation columns are key equipment in chemical separation processes used in various industries, including oil, gas, and chemicals. The design and construction of these columns on a laboratory and pilot scale involve specific stages, which are discussed below.
-
Initial Design:
- Process Analysis: Engineers first analyze the process requirements and gather data on material composition, temperature, pressure, and flow rate.
- Column Selection: Based on the type of materials and separation requirements, the type of column (such as continuous or batch distillation columns) is selected.
-
Simulation:
- Modeling: Simulation software such as Aspen Plus or HYSYS is used to predict the behavior of the distillation column under various conditions. These simulations help engineers determine optimal parameters like the number of trays and tray type.
- Performance Analysis: Simulation results are used to analyze the efficiency and yield of the distillation process.
-
Prototype Construction (Laboratory Scale):
- Small-Scale Column Construction: A small-scale prototype of the distillation column is constructed. This column is typically made of transparent or semi-transparent materials to facilitate observation of internal processes.
- Column Components: The column components include trays, packing, and feed and product collection systems.
-
Testing and Evaluation:
- Controlled Testing: The prototype is subjected to various tests. Temperature, pressure, and flow rate are carefully controlled to evaluate the column’s performance under different conditions.
- Data Collection: Data on distillation efficiency, separation yield, and product quality is collected.
-
Final Design:
- Modifications Based on Results: After analyzing the data, the final design of the column is revised considering optimization needs and feedback obtained from the tests.
-
Pilot-Scale Construction:
- Large-Scale Column Construction: The distillation column is constructed on a pilot scale. This equipment is designed to be similar to the final full-scale version.
- Infrastructure Development: The necessary infrastructure for supplying, controlling, and collecting materials is provided at this stage.
-
Real-World Testing:
- Operational Testing: The pilot-scale column is subjected to real-world operating conditions. These tests evaluate the column’s performance on a large scale and identify potential challenges.
- Feedback and Modifications: The results of these tests help engineers identify and rectify any potential problems.
The design and construction of distillation columns on a laboratory and pilot scale is a complex and precise process that allows engineers to prepare columns for large-scale production with greater confidence. This process involves design, simulation, construction, testing, and modification stages to optimize the performance and efficiency of distillation columns
Design and Construction of Reactors on a
Semi-Industrial and Laboratory Scale
1. Reactor Design:
- Reactor Type Selection: Choosing the appropriate reactor type (fixed bed, fluidized bed, CSTR, batch) based on reaction characteristics.
- Design Calculations: Performing calculations to determine reactor size, material requirements, and operating conditions.
- Component Design: Designing individual components such as the reactor vessel, stirrers, and heat exchangers.
2. Design Software:
- CAD Software: Using computer-aided design tools like AutoCAD, SolidWorks, and ANSYS for 2D and 3D modeling.
- Process Simulation Software: Employing software like Aspen Plus, COMSOL Multiphysics, and ChemCAD for simulating reactor behavior.
3. Reactor Construction:
- Material Selection: Choosing materials resistant to heat, corrosion, and other operating conditions.
- Fabrication: Using techniques like cutting and welding to create reactor components.
- Assembly: Assembling the reactor and installing auxiliary equipment.
- Testing: Conducting tests to ensure the reactor is leak-proof and functions as designed.
4. Testing and Validation:
- Performance Testing: Evaluating the reactor’s efficiency in carrying out chemical reactions.
- Verification: Ensuring the reactor meets safety and environmental standards.
- Documentation: Creating detailed records of the design, construction, and operation of the reactor.
Design and Construction of Semi-Industrial and Laboratory-Scale Mixers
Mixers are essential tools in industrial and laboratory processes, used to homogenize and blend various materials. The design and construction of semi-industrial and laboratory-scale mixers is crucial due to their importance in improving product quality and process efficiency
1. Mixer Design
1.1. Mixer Type Selection
- Blade Mixer: Suitable for low to medium viscosity materials.
- Screw Mixer: For high viscosity materials and dry mixtures.
- Rotary Mixer: For mixtures with larger, bulkier particles.
- Magnetic Stirrer: Suitable for small-scale laboratory mixtures. 1.2. Design Calculations
- Mixing Speed: Determining the appropriate speed to prevent unwanted turbulence.
- Power Requirement: Calculating the required motor power to drive the blades or screws.
- Material Viscosity: Determining the viscosity of the materials to select the appropriate blade or screw type and size. 1.3. Component Design
- Blades and Screws: Designing the shape and size of blades or screws to enhance mixing efficiency.
- Mixer Body: Designing the dimensions and materials of the mixer body to resist wear and corrosion.
- Power Transmission System: Designing the power transmission system to ensure efficient power transfer to the blades.
2. Design Software
2.1. CAD (Computer-Aided Design) Software
- AutoCAD: For drawing 2D and 3D equipment designs.
- SolidWorks: For 3D modeling and finite element analysis of components and systems.
- ANSYS: For stress analysis and fluid flow simulation in mixers. 2.2. Process Simulation Software
- Aspen Plus: For simulating chemical processes and optimizing design parameters.
- COMSOL Multiphysics: For multiphysics simulation and simultaneous analysis of flow, heat transfer, and chemical reactions.
- FLUENT: For computational fluid dynamics (CFD) simulation and analysis of complex flows in mixers.
3. Mixer Construction
3.1. Material Selection
- Wear and Corrosion Resistant Materials: Using materials like stainless steel or chemically resistant polymers.
- Lightweight and Strong Materials: Selecting materials that are both strong and lightweight for easy handling and installation. 3.2. Fabrication Process
- Cutting and Welding: Using precise cutting and welding techniques to fabricate components.
- Assembly: Assembling fabricated components with auxiliary equipment like motors and power transmission systems.
- Performance Testing: Conducting performance tests to ensure efficient and homogeneous mixing.
4. Testing and Validation
4.1. Performance Testing
- Mixing Tests: Conducting mixing tests to evaluate the mixer’s efficiency in homogenizing materials.
- Stress Testing: Assessing the mixer’s performance under various conditions. 4.2. Validation
- Compliance with Standards: Ensuring the mixer complies with environmental and safety standards.
- Documentation: Creating detailed documentation including design drawings, material specifications, and operating instructions.
Design and Construction of Laboratory-Scale Scrubbers
Scrubbers are essential tools for reducing air pollution generated from industrial processes. They are capable of removing particulate matter and toxic gases from gas streams and are widely used in the chemical, metal, and oil and gas industries
1. Scrubber Type Selection
Scrubbers are primarily categorized into two types: wet scrubbers and dry scrubbers. Wet scrubbers use liquids to absorb pollutants, while dry scrubbers employ solid sorbents.
2. Scrubber Design
- Needs Assessment: The first step in scrubber design is to assess the specific process requirements. These include the type of pollutants, gas flow rate, and operating conditions such as temperature and pressure.
- Material Selection: Selecting corrosion-resistant and abrasion-resistant materials for the scrubber body. For example, stainless steel or chemically resistant plastics.
- Dimensions and Structure: Designing the dimensions and structure of the scrubber based on precise engineering calculations. This involves determining the scrubber’s diameter and height, as well as the placement of nozzles and inlets.
3. Calculations and Simulation
- Absorption Rate: Calculating the absorption rate of pollutants using chemical and dynamic equations.
- 3D Modeling: Creating a 3D model of the scrubber using CAD software such as SolidWorks and AutoCAD.
- Process Simulation: Conducting computational fluid dynamics (CFD) simulations using software like ANSYS Fluent to optimize flow and scrubber performance.
4. Construction and Assembly
- Cutting and Welding: Precisely cutting and welding components.
- Final Assembly: Assembling components along with the installation of auxiliary equipment like pumps and nozzles.
- Leak Testing: Conducting leak tests to ensure there are no leaks in the system.
5. Testing and Startup
- Performance Testing: Conducting performance tests to evaluate the scrubber’s efficiency under actual operating conditions.
- Adjustment and Calibration: Adjusting and calibrating equipment to ensure optimal performance.
Designing and constructing laboratory-scale scrubbers is a complex, multi-step process that requires precise engineering knowledge and the use of advanced simulation and design software. By following these steps, high-efficiency scrubbers with a long service life can be produced
Design and Construction of Semi-Industrial and Laboratory-Scale Cyclones
Cyclones are one of the most important pieces of equipment for separating suspended particles from gas streams. Due to their high efficiency and simple structure, they are widely used in various industries such as mining, chemical, and food.
1. Operating Principles of Cyclones
Cyclones operate based on centrifugal force. When a gas stream enters the cyclone body, it spirals downward. This spiral motion causes the suspended particles to collide with the cyclone walls and fall to the bottom, while the clean gas exits from the top.
2. Cyclone Selection
The selection of a cyclone type depends on the process requirements and the characteristics of the suspended particles. Cyclones are generally categorized into two types:
- High-efficiency cyclones: For fine and lightweight particles.
- Medium-efficiency cyclones: For larger and heavier particles.
3. Cyclone Design
- Dimension Selection: Selecting the appropriate diameter and height of the cyclone based on the gas flow rate and particle size.
- Velocity Calculations: Determining the inlet and outlet gas velocities to optimize particle separation.
- Material Selection: Using materials resistant to wear and corrosion, such as stainless steel or resistant polymers.
4. Design Software
- SolidWorks: For 3D modeling and structural analysis.
- ANSYS Fluent: For simulating gas flow and cyclone performance using computational fluid dynamics (CFD).
- AutoCAD: For designing 2D and 3D drawings of the cyclone.
5. Cyclone Construction and Assembly
- Cutting and Welding: Using laser cutting and precise welding techniques to construct the cyclone body.
- Component Assembly: Assembling components along with the installation of auxiliary equipment such as inlet and outlet nozzles.
- Leak Testing: Conducting leak tests to ensure there are no leaks in the cyclone.
6. Testing and Evaluation
- Separation Testing: Evaluating the cyclone’s efficiency in separating suspended particles under actual operating conditions.
- Adjustment and Optimization: Adjusting operating parameters to improve cyclone performance.
Cyclones are efficient tools for separating suspended particles from gas streams, and their design and construction require precision and technical expertise. The use of design and simulation software enhances the efficiency and performance of cyclones, ensuring that the produced equipment meets environmental and safety standards.
Conclusion
The design and construction of semi-industrial and laboratory-scale process equipment is a complex, multi-step process that requires in-depth engineering knowledge and familiarity with modern tools and software. In these articles, we explored the design and construction of scrubbers, cyclones, mixers, and reactors.
Design and Simulation Software: The use of CAD software such as AutoCAD and SolidWorks for 3D modeling and simulation software like ANSYS Fluent, Aspen Plus, and Aspen Hysys for dynamic simulation and process optimization is of paramount importance.
Testing and Validation: All equipment must undergo rigorous performance and leak testing after construction to ensure correct operation and compliance with environmental and safety standards.
Ultimately, the design and construction of this equipment require not only advanced technical and engineering knowledge but also meticulous attention to detail and the use of modern techniques and tools. By following these guidelines, efficient and reliable process equipment can be produced on a semi-industrial and laboratory scale, which can be beneficial for both research and development, as well as improving industrial processes